
Rice Institute for Biomedical Research
Department of Molecular Biosciences
Northwestern University
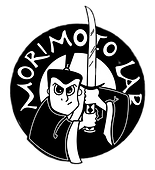
Proteostasis Collapse in Aging and Neurodegenerative Diseases

Our studies showing age-dependent aggregation and proteotoxicity benefitted from the development of C. elegans transgenic lines expressing polyQ and mutant SOD1 proteins in body wall muscle cells, intestine and neurons (Satyal et al 2000; Morley et al 2002; Brignull et al 2006; Gidalevitz et al., 2009; Sinnige et al., 2021).
Jim Morley made the initial key observation linking proteostasis with aging showing that the Q35::YFP reporter lines exhibited a striking age-dependence for both aggregation and toxicity in a polyQ-length dependent manner, and that these phenotypes were regulated by the insulin-signaling (ILS) pathway, a potent regulator of lifespan, and was dependent upon DAF-16 and HSF-1 (Morley et al 2002; Brignull et al 2006; Teixeira-Castro et al 2011; Morley and Morimoto 2004). Consistent with these results, genetic knockdown of HSF-1 not only strongly promoted aggregation but completely suppressed the longevity benefits of the insulin-signaling pathway.
Anat Ben-Zvi then demonstrated that the HSR in C. elegans declined in aging and was associated with aggregation and loss-of-function of endogenous temperature-sensitive reporter proteins in during aging at the permissive temperature (Ben-Zvi et al 2009). Expression of expanded polyQ or mutant SOD1 was shown to cause misfolding of TS-mutant proteins revealing that misfolding is amplified by certain aggregation-prone proteins and the effects of aging (Gidalevitz et al 2006; 2009; 2010). We suggested that the capacity of the proteostasis network to prevent misfolding of endogenous metastable proteins is compromised during aging, and that the age-associated decline in the proteostasis network is likely due to the loss in robustness of cell stress responses leading to the reduced capacity of chaperones and clearance machines.
John Labbadia demonstrated that the age-dependent decline in organismal proteostasis and the heat shock response is regulated by germline stem cells and that the heat shock response was fully restored when germline signaling was blocked (Labbadia and Morimoto, 2015). This link between aging and reproduction was recently elaborated by Ambre Sala who used a genetic screen to demonstrate that damage to the vitelline layer of the eggshell of the fertilized egg prevents the age-dependent decline in the heat shock response, stress resilience and proteostasis (Sala et al., 2020). Both DAF-16 and HSF-1 are required in addition to signaling via the vulva which likely processes the health status of the fertilized egg to the parent.
Two lines of evidence support an important role of the proteostasis network and the heat shock response in human aging. The expression of human molecular chaperones in normal brain aging declines which was tested experimentally to identify those chaperones important to suppress mutant Huntingtin aggregation in human cells or aggregation of Abeta and polyQ in C. elegans (Brehme et al., 2014). The candidate genetic screen identified the same core chaperome of 18 genes of the Hsp70, Hsp90, J-domain, CCT and TPR-containing co-chaperones in C. elegans and human cells, all of which declined in brain aging. Another line of support that proteostasis declines in human aging follows from the role of the NAD-dependent sirtuin, SiRT1, in the regulation of the HSR (Westerheide et al 2009). Sandy Westerheide studied the attenuation of the HSR and found that HSF-1 is acetylated in the DNA binding domain by p300/CBP, thus preventing binding of HSF-1 at the heat shock elements in promoter region of heat shock genes. The deacetylase, SiRT1, maintains HSF-1 in a DNA binding-competent state and thus determines the persistence of HSF-1 transcriptional activity. These results showed that HSF-1 activity can be enhanced using a regulatory override strategy of modulating the cellular levels of NAD or the balance of acetyltransferases and SiRT1, thus prolonging the “youthfulness” benefit of stress resilience promoted by HSF-1 and robustness of the heat shock response.
​
References
Brehme, M., C. Voisine, T. Rolland, S. Wachi, J. Soper, Y. Zhu, K. Orton, A. Villella, D. Garza, M. Vidal, H. Ge, and R.I. Morimoto. A Conserved Chaperome Sub-Network Safeguards Protein Homeostasis in Aging and Neurodegenerative Disease. Cell Reports 9: 1135–1150, DOI: 10.1016/j. PMID: 25437566 (2014).
Brignull, H.R., J.F. Morley, S.M. Garcia, and R.I. Morimoto. Modeling Polyglutamine Pathogenesis in C. elegans. Methods in Enzymology 412: 256-282. (2006).
Brignull, H., F. Moore, S. Tang, and R. I. Morimoto. Polyglutamine Proteins at the Pathogenic Threshold Display Neuron-Specific Aggregation in a Pan-Neuronal Caenorhabditis elegans Model. The Journal of Neuroscience 26(29): 7597-7606. (2006).
Finkbeiner, S., A.M. Cuervo, R.I. Morimoto and P.J. Muchowski. Disease-modifiying pathways in neurodegeneration. The Journal of Neuroscience 26(41): 10349-10357. (2006).
Garcia, S. M., M.O. Casanueva, M.C. Silva, M.D. Amaral, and R.I. Morimoto. Neuronal signaling modulates protein homeostasis in C. elegans postsynaptic muscle cells. Genes and Development 21: 3006-3016. (2007).
Gidalevitz, T., A. Ben-Zvi, K. Ho, H. Brignull, and R. I. Morimoto. Progressive Disruption of Cellular Protein Folding in Models of Polyglutamine Diseases. Science 311: 1471-1474. (2006).
Gidalevitz, T., E.A. Kikis, and R.I. Morimoto. A Cellular Perspective on Conformational Disease: The Role of Genetic Background and Proteostasis Networks. Current Opinions in Structural Biology 20: 23-32. (2010).
Gidalevitz, T., T. Krupinski, S.M. Garcia, and R.I. Morimoto. Toxicity of Mutant SOD1 is Directed by Protein Polymorphisms. PLoS Genetics 5(3): e1000399. (2009).
Gidalevitz, T., V. Prahlad, and R. I. Morimoto. The Stress of Protein Misfolding: From Single Cells to Multicellular Organisms. Cold Spring Harbor Perspectives in Biology, 3: PMID: 21536706. (2011).
Gidalevitz, G., N. Wang, T. Deravaj, and R.I. Morimoto. Natural Genetic Variation Determines Susceptibility to Aggregation or Toxicity in a C. elegans model for Polyglutamine Disease. BMC Biology 11: 100. DOI: 10.1186, PMID: 24079614 (2013).
Holmberg C.I., Hietakangas V., Mikhailov A., Rantanen J.O., Kallio M., Meinander A., Hellman J., Morrice N., MacKintosh C., Morimoto R.I., Eriksson J.E., Sistonen L. Phosphorylation of serine 230 promotes inducible transcriptional activity of heat shock factor 1. EMBO, 20(14): 3800-3810. (2001).
Kikis, E.A., A. Ben-Zvi, and R.I. Morimoto. C. elegans as a Model System to Study the Biology of Protein Aggregation and Toxicity. In: Protein Misfolding Diseases: Current and Emerging Principles and Therapies (Edited by M. Ramirez-Alvarado, J. Kelly, and C. Dobson), p. 175-190, Wiley Press. (2010).
Kitamura, A., H. Kubota, C. Pack, G. Matsumoto, S. Hirayama, Y. Takahashi, H. Kimura., M. Kinjo, R. I. Morimoto and K. Nagata. Cytosolic Chaperonin Prevents Polyglutamine Toxicity with Altering the Aggregation State. Nature Cell Biology 8(10): 1163-1175. (2006).
Krammer, C., K.W. Park, L. Li, R. Melki, and R.I. Morimoto. Spreading of a Prion Domain from Cell to Cell by Vesicular Transport in C. elegans. PLoS Genetics 9(3): DOI: 10.1371, PMID: 23555277. (2013).
Labbadia, J., and R.I. Morimoto. Huntington’s Disease – Underlying Molecular Mechanisms and Emerging Concepts. Trends in Biochemical Sciences 38(8): 378-385. DOI:10.1016, PMID: 23768628 (2013).
Matsumoto, G., S. Kim, and R.I. Morimoto. Huntingtin and mutant SOD1 form aggregate structures with distinct molecular properties in human cells. J Biol Chem. 281: 4477-4485. (2006).
Matsumoto G., Stojanovic A., Holmberg C.I., Kim S., Morimoto R.I. Structural properties and neuronal toxicity of amyotrophic lateral sclerosis-associated Cu/Zn superoxide dismutase 1 aggregates. Journal of Cell Biology, 171(1): 75-85. (2005).
Morley J.F., Brignull H.R., Weyers J.J., Morimoto R.I. The threshold for polyglutamine-expansion protein aggregation and cellular toxicity is dynamic and influenced by aging in Caenorhabditis elegans. Proc Natl Acad Sci U S A.99(16):10417-22. (2002).
Morley J.F. and Morimoto R.I. Regulation of longevity in C. elegans by heat shock factor and molecular chaperones. MBC in Press, published December 10 as 10.1091/mbc.E03-07-0532. (2003).
Morimoto, R.I. Proteotoxic Stress and Inducible Chaperone Networks in Neurodegenerative Disease and Aging. Genes and Development 22: 1427-1438. (2008).
Morimoto, R.I. Stress, Aging and Neurodegenerative Disease. The New England Journal of Medicine 355(21): 2254-2255. (2006).
Nollen EA, Garcia SM, van Haaften G, Kim S, Chavez A, Morimoto RI, Plasterk RH. Genome-wide RNA interference screen identifies previously undescribed regulators of polyglutamine aggregation. Proc Natl Acad Sci U S A, 101: 6403-6408. (2004).
Prahlad, V. and R.I. Morimoto. Neuronal Circuitry Regulates the Response of C. elegans to Misfolded Proteins. Proc. Natl. Acad. Sci. U.S.A. 108: 14204-14209. PMID: 1106557108. (2011).
Prahlad, V., and R.I. Morimoto. Integrating the Stress Response: Lessons for Neurodegenerative Diseases from C. elegans. Trends in Cell Biology 19: 52-61. (2009). PMID: 19112021 [PubMed – as supplied by publisher]
Rieger TR, Morimoto RI, and V. Hatzimanikatis. Bistability explains threshold phenomena in protein aggregation both in vitro and in vivo. Biophys J. 90: 886-95. (2006).
Rieger, T., R.I. Morimoto, and V. Hatzimanikatis. Mathematical Modeling of the Eukaryotic Heat Shock Response: Dynamics of the Hsp70 Promoter. Biophysical Journal 88: 1646-1658. (2005).
Satyal SH, Schmidt E, Kitagawa K, Sondheimer N, Lindquist S, Kramer JM, Morimoto RI. Polyglutamine aggregates alter protein folding homeostasis in Caenorhabditis elegans. Proc Natl Acad Sci U S A 23;97(11): 5750-5. (2000).
Shen X, Ellis RE, Lee K, Liu CY, Yang K, Solomon A, Yoshida H, Morimoto R, Kurnit DM, Mori K, Kaufman RJ. Complementary signaling pathways regulate the unfolded protein response and are required for C. elegans development. Cell 107: 893-903. (2001).
Sinnige, T., G. Meisl, T. C. T. Michaels, M. Vendruscolo, T. P.J. Knowles, and R. I. Morimoto. Kinetic Analysis Reveals that Independent Nucleation Events Determines the Progression of Protein Aggregation in C. elegans. Proc. Natl. Acad. Sci. U.S.A. (in press, 2021).
Soojin Kim, Ellen A. A. Nollen, Kazunori Kitagawa, Vytautas P. Bindokas and Richard I. Morimoto. Polyglutamine protein aggregates are dynamic. Nature Cell Biology, 4: 826-831. (2002).
Teixeira-Castro, A., M. Ailion, A. Jalles, H. R. Brignull, J. L. Vilaça, N. Dias, P. Rodrigues, J. F. Oliveira, A. Neves-Carvalho, R. I. Morimoto, and P. Maciel. Neuron-Specific Proteotoxicity of Mutant Ataxin-3 in C. elegans: Rescue by the DAF-16 and HSF1 Pathways. Human Molecular Genetics 20(15): 2996-3009. PMID:21546381 (2011).
Yu, A., S.G. Fox, A. Cavallini, C. Kerridge, M. J. O’Neill, J. Wolak, S. Bose, and R. I. Morimoto. Tau Protein Aggregates Inhibit the Protein-Folding and Vesicular Trafficking Arms of the Cellular Proteostasis Network. J. Biological Chemistry 294(19): 7917-7930. DOI:10.1074/jbc.RA119.007527. (2019).
Yu, A., Y. Shibata, B. Shah, B. Calamini, D. Lo, and R.I. Morimoto. Protein Aggregation Inhibits Clathrin-Mediated Endocytosis by Chaperone Competition. Proc. Natl. Acad. Sci. USA 111: E1481-1490. DOI: 10.1073/pnas.1321811111, PMID: 24706768. (2014).
​
​
​